Stereotactic Radiosurgery – SRS and SBRT Techniques
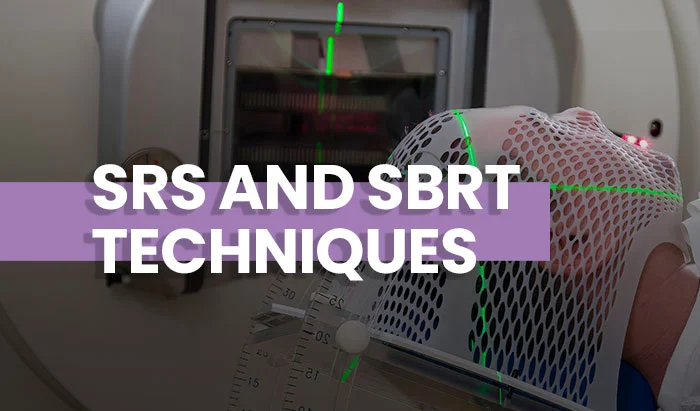
Introduction
Radiation therapy is one of the most common methods used to treat cancer. It involves the use of high-energy radiation to destroy cancer cells. One of the radiation therapy techniques used is stereotactic radiosurgery (SRS). SRS is a precise radiation treatment method created for the treatment of smaller-sized tumors and anomalies within the brain. The material science and dosimetric standards of intracranial stereotactic radiosurgery give high-precision and high-dose delivery inside the tumor. SRS is a smaller-sized non-surgical method that conveys precisely targeted, high-dose radiation in a single or exceptionally few treatment fractions, as compared to conventionally-fractionated radiotherapy. The advancement of medical imaging and radiation therapy technologies is the basis of the evolution of stereotactic radiosurgery, which allows treatment with a maximum dose deposition in the target while minimizing radiation damage to the surrounding critical organs.
Historical Overview
Today, we call non-surgical radiation therapy performed on an outpatient basis stereotactic radiosurgery, used to treat functional abnormalities and small tumors of the brain. But in some cases, stereotactic radiosurgery can deliver precisely-targeted radiation high-dose treatments than traditional therapy, which can help avoid healthy tissue, and damage the DNA of tumor cells; as a result, these cells lose their ability to reproduce within a limited number of fractions. Furthermore, when SRS is used to treat body tumors, it’s called stereotactic body radiotherapy (SBRT).
Stereotactic Radiosurgery Procedure and Equipment
SRS is a highly specialized procedure that involves a team of medical professionals with specialized skills. The team typically includes a radiation oncologist, medical radiation physicist, radiologist, dosimetrist, radiation therapist, and radiation therapy nurse.
Stereotactic radiosurgery (SRS) and stereotactic body radiation therapy (SBRT) rely on several technologies comparable to 3D conformal and inverse planning such as three-dimensional imaging techniques, X-rays, and highly focused gamma, or proton beams that converge on a tumor or abnormality. Like any Inverse planning, diagnostic imaging is used to help find the tumor and determine which area will be treated. This includes four-dimensional imaging, which tracks the target area’s movement over time as the patient breathes. Before images are taken, gold seeds called fiducials are occasionally injected into the tumor as the only invasive element of the treatment. Physicians employ the fiducials to guarantee that the high-dose envelope of radiation is correctly covering the tumor because they are visible in planning scans and during treatment. Radiation oncologists collaborate with medical physicists to create a radiation strategy that protects normal structures from harm. Unlike standard inverse planning, however, SBRT sessions last 30 minutes to one hour, the number of fractions is limited, and the energy output is much higher.
SRS and SBRT can be delivered using a linear accelerator or Gamma Knife. These two types involve the following phases: placement of the head frame, imaging of the tumor location, computerized dose planning, quality assurance of the positioning, and radiation delivery. Proton radiation is used for larger, more irregularly shaped lesions.
Figure 1: LINAC room
Figure 1 shows the radiotherapy room where the SRS and SBRT treatments can be delivered using the same linear accelerator as IMRT or VMAT techniques, using higher energy and different methods of planning.
A high dose going to 120% of the prescription is accepted inside the target, the physicist considers the targets as constraints and the organ at risk as an objective to improve the coverage homogeneity inside this target. As well, the sequencing parameters and calculation properties will be changed to cover small fields with accuracy. The treatment itself only takes around 20-40 minutes.
Figure 2: Gamma knife room (Source: https://www.elekta.com)
Figure 2 shows the gamma knife device that bundles approximately 200 rays from radioactive sources (cobalt 60) by collimation. The patient is treated for only one session by positioning his head in such a way that the isocenter overlaps with the tumor of the patient. In this position, the patient remains fixed by a stereotactic frame until the therapeutic dose is reached according to the dose distribution plan. Because of the high dose gradient, the organs at risk will be protected from doses above their radiation tolerance level. The gamma knife is used for pathologies of the head with a diameter of less than 3 centimeters. The treatment time varies between 30 minutes and 3 hours depending on the size, shape, and location of the lesion and the number of radiation doses.
LINAC technology is much more common than Gamma Knife technology because it is more practical since the gantry rotates around the patient to deliver the radiation from different angles. In addition, a more routine pre-planning practice with LINAC-based stereotactic radiosurgery is that the MRI can be achieved before the frame placement, and then a CT with the frame in place is commonly acquired.
These two techniques have some limitations: for larger, more irregularly shaped lesions, it becomes more difficult to deliver a uniform dose of radiation to the target without affecting outlying tissue. In these cases, proton radiation is used.
Figure 3 : Proton beam therapy (https://doi.org)
Figure 3 shows Proton beam therapy which is a charged particle radiosurgery, the newest type of stereotactic radiosurgery and is available in only a few research centers in the U.S, although the number of centers offering proton beam therapy has greatly increased in the last few years. Proton beam therapy can treat brain cancers in a single session using stereotactic radiosurgery, or it can use fractionated stereotactic radiotherapy to treat body tumors over several sessions.
SRS and SBRT Advantages
SRS and SBRT offer several advantages over traditional surgery and radiation therapy methods. They are important alternatives to invasive surgery, especially for patients who are unable to undergo surgery and for tumors and abnormalities that are located close to vital organs or are hard to reach, as well as the organs moving within the body.
Stereotactic radiosurgery is used to treat different types of brain tumors including benign and malignant tumors in different stages – primary (Figure 4) and metastatic (Figure 5).
Figure 4: Primary brain tumor
Figure 5: Brain metastasis
In some cases, stereotactic radiosurgery is used to treat residual tumor cells following surgery and arteriovenous malformations (AVMs). SBRT is currently used to treat malignant or benign small-to-medium-size tumors in other disease sites, including the lung, abdomen, spine, prostate, and head and neck.
Figure 6: Lung tumor
Figure 7: Pancreas tumor
Figure 6 shows a dose distribution in the lung target, there is concern about treating a moving target with a highly modulated beam. Figure 7 shows the dose distribution inside the pancreas. As well, it is hypothesized that the delivery of ablative doses to bone metastases may improve rates of pain response and duration, alongside greater local control. Several reviews have been published reporting the feasibility and efficacy of SBRT for bone metastases. For SRS and SBRT treatment there are different tolerances used for all the critical organs.
Additional Requirements
During stereotactic radiosurgery treatment, a neurologist or neuro-oncologist may participate with the radiation oncologist and neurosurgeon in the multidisciplinary team that considers various treatment options for individual cases and helps decide who may benefit from radiosurgery for lesions in the brain.
Stereotactic radiosurgery treatments require a high level of precision. Fixation equipment such as a head frame (figure 9) or a plastic mask (figure 10) are necessary to ensure treatment efficiency. Headframes can be very uncomfortable and invasive for the patient. Nonetheless, LINAC-based treatments are moving towards frameless SRS, notably using the head plastic mask to immobilize the head.
Figure 8: Headframe https://www.itnonline.com
Figure 9: Head plastic mask
For SBRT, using a LINAC, typically one to five treatment sessions are required and are fractionated throughout one to two weeks. After the simulation and immobilization, a 4DCT is an additional requirement to visualize the tumor movement while breathing; this case is very common for tumors in the lungs or liver. Moreover, X-rays will be taken during the treatment to monitor the position of the tumor. Image-guided radiation therapy (IGRT) is used to confirm the location of the tumor immediately before, and in some cases, during the delivery of radiation. IGRT improves the precision and accuracy of the treatment.
Finally, SRS and SBRT require a higher degree of quality assurance and tighter linear accelerator tolerances due to smaller fields and variations in dose rate while the gantry rotates during treatment delivery.
Future Directions
Advancements in technology have made it possible to improve the efficiency and effectiveness of SRS and SBRT treatments. For example, the development of linear accelerator-based systems has made it possible to deliver high doses of radiation to tumors more precisely and with less damage to surrounding healthy tissue. In addition, the use of image guidance systems has made it possible to monitor the position of tumors during treatment, further improving the accuracy of the treatment.
The future of SRS and SBRT is bright, with ongoing research and development aimed at improving the efficiency and effectiveness of these treatments. Researchers are exploring new ways to deliver radiation to tumors, such as using nanoparticles or other targeted delivery methods. In addition, there is ongoing research into the use of SRS and SBRT for a wider range of cancers, including breast cancer, liver cancer, and pancreatic cancer.
Another area of research is focused on improving the quality of life for cancer patients undergoing radiation therapy. This includes developing new ways to manage side effects, such as fatigue and nausea, as well as developing new ways to monitor patients during treatment to ensure that they are receiving the correct dose of radiation.
Conclusion
In conclusion, SRS and SBRT are valuable additions to the range of treatments available for cancer patients. These treatments offer a high degree of precision and accuracy in delivering radiation to tumors, while minimizing damage to surrounding healthy tissue. As technology continues to advance and treatments continue to be refined, the use of SRS and SBRT is likely to become even more widespread, offering hope to patients who were previously considered untreatable.
Because of the greater complication rates, patients with tumors that are centrally situated or adjacent to the airways or the heart have been deemed poor candidates for SBRT, but recent studies showed that these treatments are doable using slightly lower doses of radiation in combination with radiofrequency ablation. In the last 2 years, SBRT had a success rate of 80 to 90 percent, which is comparable to resection surgery but with significantly less complications.
While SRS and SBRT are not suitable for all patients or all types of tumors, they offer a valuable addition to the range of treatments available for cancer patients. As technology continues to evolve and techniques continue to be refined, the use of SRS and SBRT is likely to become even more widespread, offering hope to patients who were previously considered untreatable.
References
Understanding the different variations is immensely helpful to clinicians planning interventional procedures to treat coronary artery disease after diagnosis, particularly when there are secondary changes of calcification, plaque formation, and stenosis. Treatments for coronary artery disease can include:
- https://www.britannica.com
- https://www.mayoclinic.org
- https://www.cancer.gov
- https://www.uclahealth.org
- https://pubmed.ncbi.nlm.nih.gov
- https://www.aans.org
- https://pubmed.ncbi.nlm.nih.gov
- https://www.ncbi.nlm.nih.gov
- https://www.aapm.org
- Applications of stereotactic radiosurgery in neuro-oncology. Isabela Pena Pino 1, Kunal S. Patel 2, Paul H. Chapman 3, Marc Bussiere 4, Jay S. Loeffler 4, Clark C. Chen 1 – https://doi.org
Disclaimer: The information provided on this website is intended to provide useful information to radiologic technologists. This information should not replace information provided by state, federal, or professional regulatory and authoritative bodies in the radiological technology industry. While Medical Professionals strives to always provide up-to-date and accurate information, laws, regulations, statutes, rules, and requirements may vary from one state to another and may change. Use of this information is entirely voluntary, and users should always refer to official regulatory bodies before acting on information. Users assume the entire risk as to the results of using the information provided, and in no event shall Medical Professionals be held liable for any direct, consequential, incidental or indirect damages suffered in the course of using the information provided. Medical Professionals hereby disclaims any responsibility for the consequences of any action(s) taken by any user as a result of using the information provided. Users hereby agree not to take action against, or seek to hold, or hold liable, Medical Professionals for the user’s use of the information provided.