MRI in Multiple Sclerosis (MS): Protocols and Best Practices
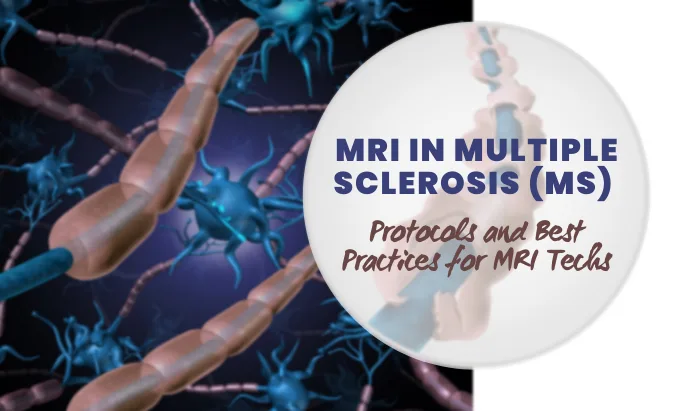
MRI in Multiple Sclerosis (MS): Protocols and Best Practices
Everything you need to know about the MRI protocols (both brain and spinal cord) for MS, including best practices for strict standardization, technical considerations and parameters, the use of contrast media, and more
Since there is no definitive test for multiple sclerosis (MS), patients suspected of having the disease undergo a variety of different tests in diagnostic process. Magnetic resonance imaging (MRI) is part of that process. In this article, we’ll cover what you as an MRI technologist need to know about MRI for MS, including recommended protocols for different areas of interest (such as the brain) and associated technical considerations and details, the use of gadolinium-based contrast agents, and unconventional MRI sequences for imaging multiple sclerosis.
- MRI Protocols for Multiple Sclerosis (MS) Overview
- Role of Radiology in Diagnosing MS
- Standardization of MRI Protocols for MS
- Gadolinium-Based Contrast Agents
- Non-Conventional MRI Sequences in MS
- Improve Patients’ MRI Experience
- A Quick Summary
- References
MRI Protocols for Multiple Sclerosis (MS) Overview
Multiple sclerosis (MS) is an autoimmune chronic demyelinating disease of the central nervous system (CNS). Despite many years of research, the cause of MS remains unknown. Its development, however, is influenced by a combination of genetic, immunological, and environmental factors. MS affects both the brain and the spinal cord, causing a wide variety of neurological symptoms that vary in type and severity[1]. Patients with MS are most often affected by fatigue, pain, bladder and bowel issues, vision problems, cognitive fog, and emotional changes[2].
Diagnosing MS
Other causes of neurological symptoms should be ruled out before making a definitive MS diagnosis. There is no specific test for MS, so an exhaustive medical history associated must be taken, along with a thorough neurological exam. And battery of tests should also be considered, including a blood test, checking for specific biomarkers associated with MS, a lumbar puncture for identifying abnormalities in MS-associated antibodies, an evoked potential test to measure the speed at which the information travels down the patient’s nerve pathways, and an MRI scan to reveal any damage or scarring of the myelin sheath in the patient’s brain and spinal cord [3].
The gist: MS is an inflammatory demyelinating disease whose diagnosis is based on a battery of clinical, biological, and radiological tests.
Role of Radiology in Diagnosing MS
MRI has become a routine and essential tool for diagnosing MS. It also plays a major role in assessing the progression of the disease, monitoring treatment efficacy, and safety. It even contributes to our understanding of the natural history of MS[2]. In fact, when performed correctly, MRI can give us critical information about disease activity, which is exactly what led its findings to being included in the McDonald criteria for MS diagnosis that were first established in 2001 by the international panel on the diagnosis of MS[4]. The McDonald criteria incorporate clinical and imaging data and require objective evidence of CNS damage (white matter lesions) that is spread out over in time (occurring on different dates) and found in different parts of the CNS)[3-5]. The 2017 revision to the McDonald criteria offers a better understanding of MS and allows for an earlier diagnosis, which in turn provides patients with access to the right treatment sooner[6]. Moreover, MRI’s ability to characterize disease burden and detect microstructural tissue alterations has greatly improved with the introduction of modern quantitative MR techniques, such as diffusion tensor imaging (DTI), magnetization transfer imaging (MTI), and proton spectroscopy[2].
The gist: MRI’s ability to detect white matter lesions and follow their dissemination in time and space is essential for diagnosing MS.
Standardization of MRI Protocols for MS
Once a definite MS diagnosis has been clearly established, MRI scans are no longer need for diagnostic purposes. Instead, additional scans will be used to track disease activity over time through the detection of new enhanced or non-enhanced lesions. Therefore, a well-performed standardized MRI exam is key since acquisition parameters (i.e., magnetic field strength, pulse sequences, spatial resolution) can substantially influence the detection of focal MS lesions. In fact, images acquired on different machines could be difficult to compare for accurate and confident identification of new lesion activity, which is why patients are always advised to perform their MRI follow-up exams on the same scanner at the same imaging center.
The adoption of standardized MRI protocol in clinical practice in different healthcare systems and countries is a major challenge. However, a recent attempt by the Magnetic Resonance Imaging in Multiple Sclerosis (MAGNIMS)[7] and the Consortium of Multiple Sclerosis Centers (CMSC)[8], have prompted societies around the world to establish consensus guidelines on the use of MRI in MS. In 2021, an international consensus was published and provided “updated guidance on how and when to use MRI for diagnosis, prognosis, and treatment monitoring of MS, with special focus on the use of standardized MRI protocols, and the judicious use of GBCAs”[9]. The need for strict standardization of MRI acquisition and interpretation to avoid misdiagnosis cannot be overstated, especially at the first clinical presentation and during the early course of the disease since it is during these times that it provides important prognostic information.
Acquisition and Technical Considerations
The 2021 consensus recommended the use of 3T MRI machines (over the 1.5T) due to their improved resolution and signal-to-noise ratio which allow for an improved sensitivity to focal MS lesions detection. However, when compared to a 1.5T magnetic field strength, 3T machines do not have any consequences in terms of possible earlier diagnosis of MS. Additionally, 3T machines have no added value when compared to 1.5T machines for spinal cord MRIs.
For brain MRI protocols for MS, the use of 3D acquisition techniques instead of 2D is highly recommended, particularly for FLAIR and T1 sequences as they will improve lesion detection and the realignment of anatomic orientation. For 2D sequences, spatial resolution should consist of a maximum slice thickness of 3 mm and an in-plane special resolution of 1×1 mm. For spinal cord MRI protocols, slice thickness should not exceed 3 mm in sagittal acquisitions and 5 mm in axial acquisitions.
Only macrocyclic gadolinium-based contrast agents should be used with a standard doses of 0.1 mmol/kg body weight, and a minimum delay of 5–10 minutes. It is also recommended to follow the “one stop shot” strategy for spinal cord imaging in which images are directly acquired after contrast-enhanced brain imaging. This will save time and reduce the need for additional contrast administration[7].
MRI Timing
A baseline MRI scan is essential before a patient is started on or switched to a new disease-modifying treatment. A second baseline scan should also be done 3-6 months after therapy begins to avoid any misinterpretation of lesions (usually done without gadolinium, unless the first baseline enhanced T1 image is unavailable). MRI scans should be performed on an annual basis for patients receiving disease-modifying treatment.
The gist: Defining a standardized MRI protocol for the diagnosis of MS is key for a proper follow-up and cross-comparison between two different scans.
Recommended Brain MRI Protocol for MS
The following sequences are recommended for a brain MRI scan[9]:
Based on its high sensitivity, sagittal 3D FLAIR acquisition is the core sequence for MS diagnosis and monitoring[10]. Pre-contrast T1-weighted sequences are not routinely needed because pre-contrast images rarely assist with interpretation of post-contrast hyperintensities. However, pre-contrast T1-weighted sequences are very important for research purposes, especially for the quantitative assessment of brain volume[11].
Recommended Spinal Cord MRI Protocol for MS
The following sequences are recommended for a spinal cord MRI scan[9]:
Gadolinium-Based Contrast Agents
Gadolinium (Gd)-based contrast agents (GBCA) increase the signal of a target structure of the body by shortening the T1 and T2 relaxation times of its surrounding tissue. GBCA will also detect and characterize disruptions in normal physiology, i.e. abnormal permeability of tumor-associated micro-vessels, angiogenesis, and blood–brain barrier (BBB) disruption (increased extravasation of immune cells and poorly regulated flux of molecules and ions across the BBB)[12]. On T1-weighted sequences post-contrast, new lesions appear enhanced which makes them useful for diagnostic purposes as they indicate dissemination in time and for monitoring disease activity.
Until recently, GBCAs were considered safe in their application. However, a report in 2006 associated GBCA injections dissociation with the development of nephrogenic systemic fibrosis (NSF) which is a rare disorder that develops in patients with severe kidney failure[13,14]. More recently, Gd accumulation in the CNS, identified as high signal intensities (SI) in the dentate nucleus and the globus pallidus of patients with normal kidney function, was reported[15]. The chelating structure of these GBCAs was later found to be a confounding factor. Indeed, SI increases were correlated with linear rather than macrocyclic GBCAs administration[16-19]. To this end, linear GBCAs were suspended from the market while macrocyclic GBCAs use continued as no available MRI evidence linked them to brain gadolinium retention[20].
Prerequisite for a Gadolinium-Based Contrast Agent Injection
GBCAs are contraindicated in patients who previously had severe allergic reactions; patients with severe renal disease (eGFR <30 mL/min/1.73 m2) or acute deteriorating renal function; and patients who are or might be pregnant.
Gadolinium-Based Contrast Agent in MS
Even though gadolinium deposition in the CNS has been recognized by several governmental agencies, gadolinium remains invaluable especially the initial phases of MS to determine dissemination in time. When considering the standardization of the MS MRI protocol, it is very important to keep the delay between contrast administration and the T1-weighted acquisition identical during follow-up scans and not shorter than 5 minutes (ideally 10 minutes). One way to assure this delay is to inject the GBCA intravenously before the FLAIR sequence and to acquire the enhanced T1-weighted sequence at the end of the protocol.
GBCA use is recommended for diagnostic purposes to, as previously stated, show dissemination in time on a baseline MRI, contribute to differential diagnosis and predict future disease activity. GBCAs are also used to monitor disease activity if no baseline MRI was acquired (particularly in patients on interferon-Beta and glatiramer acetate). However, they are not recommended in short follow-up MRIs (within 6 months).
In summary, the use of GBCAs is optional and not recommended for all clinical situations. They should be used judiciously and limited to a single dose.
The gist: Due to the latest findings of gadolinium retention in the brain following repetitive administrations, the use of GBCA has been regulated and is limited to macrocyclic GBCA.
Non-Conventional MRI Sequences in MS
Magnetization Transfer
The magnetization transfer ratio is measured by using gradient-echo or spin-echo MR sequences with and without an off-resonance saturation pulse. When decreased, it indicates a reduction in the capacity of the protons bound to the brain tissue matrix to exchange magnetization with the surrounding free water. Consequently, an estimation of the disrupted tissue structure is provided[21].
Diffusion Tensor Imaging
Diffusion tensor imaging enables the measurement of water molecules random Brownian movement by applying diffusion-weighted magnetic field gradients to an echo-planar MR sequence. Diffusion metrics, which are sensitive to the size and geometry of water-filled spaces[21], such as mean diffusivity (MD), fractional anisotropy (FA), as well as axial (AD) and radial diffusivities (RD) will be derived from this motion measurement. Studies have shown different extents of significant MD, AD, and RD increases and FA decreases in T2-hyperintense lesions, normal-appearing white matter, and gray matter of MS patients[22,23].
Magnetic Resonance Spectroscopy
Using hydrogen 1 (1H) MR spectroscopy, studies have shown dynamic changes of metabolite concentrations in normal-appearing white matter regions that eventually become focal lesions. These changes were even reported in acute MS lesions that had been present for several months[21]. Acute MS lesions have been more specifically shown to contain higher levels of choline and lactate, which reflect an increase in the metabolism of inflammatory cells, as well as lower levels of N-acetyl-aspartate (NAA), which is a marker of neuroaxonal loss[22].
The gist: Advanced MRI techniques have improved the assessment of brain tissue integrity and enabled the characterization of disease burden.
Improve Patients’ MRI Experience
For many patients, undergoing an MRI scan is a stressful experience that may cause fear, claustrophobia, or anxiety. Having to remain still in an enclosed space throughout a long and loud acquisition process is frequently uncomfortable, if not painful. And this already stressful experience is often worsened by the practices of most radiology departments, who prioritize productivity and cost management over patient comfort. As a result, anxious patients 1) will move during the examination and cause motion artifact, 2) will not be able to complete the scan, or even 3) will not show up for the exam.
Helping patients overcome their anxiety by turning the MRI scan into a comfortable, fast, and entertaining experience will be beneficial for both the patient and the department. You can provide the patients with such an experience by introducing yourself often to the patients in the department to create a personnel connection and letting them know how long it would be until their scan. They can use humor whenever possible to lighten the patients’ mood and assist both inpatients and outpatients to the scanning table. If the patient is still anxious, a loved one can be allowed into the scan room for emotional support, and in extreme cases, sedation can be the best option. Comforting the patient while doing the scan is also key—you can tell the patient how long the scan is going to take, let them know when a long or noisy sequence is coming up, check on them to see if they are okay and tell them they are doing well, and talk to them on multiple occasions during the scan. If the machine setup allows it, you should offer your patients the option to listen to the music of their choice (provide a list of available music or ask the patients to bring their own music). Finally, remember to commend the patient for doing a “good job” or for “doing well” in the scanner.
The gist: Comfortable patient is a happy, less anxious cooperative patient. It is always best to provide your patients with as much comfort as possible during the MRI exam in order to get a better outcome—both in terms of acquisition and patient experience.
A Quick Summary
MRI plays a critical role in both diagnosing and monitoring the progression of MS, especially when protocols are strictly standardized—which makes your skill as a technologist vital to the success of the patient’s treatment plan. Patients should be scanned on the same machine whenever possible, under the same conditions, so images can be more easily compared and the progression of the disease more effectively monitored. For brain MRI scans, you should know and master sagittal 3D FLAIR acquisitions, as these are the primary sequence for diagnosing and monitoring MS. If required to use 2D sequences for brain scans, be sure to set the slice thickness to no more than 3 mm and the in-plane special resolution to 1×1 mm. For the spinal cord, slice thickness should be no more than 3 mm in sagittal acquisitions and 5 mm in axial acquisitions. When doing a scan with GBCAs, you should administer a standard dose of macrocyclic GBCA of 0.1 mmol/kg of body weight, and a set minimum delay of 5–10 minutes. The “one stop shot” strategy is recommended for spinal cord imaging in which images are directly acquired after contrast-enhanced brain imaging to save time and reduce the need for additional GBCA administration. As with all examinations, you should do your best to make patients as comfortable as possible—remember that patients may be scared, anxious, stressed, or all of the above—in order to help patients have a better experience and to increase the quality of the images you acquire, which in turn, directly contribute to the patient’s treatment and wellbeing.
References
- Confavreux, C., & Vukusic, S. (2014). The clinical course of multiple sclerosis. Handbook of Clinical Neurology, 122, 343–369. [Link]
- Filippi, M., Bar-Or, A., Piehl, F., Preziosa, P., Solari, A., Vukusic, S., & Rocca, M. A. (2018). Multiple sclerosis. Nature Reviews. Disease Primers, 4(1), 43. [Link]
- Polman, C. H., Reingold, S. C., Banwell, B., Clanet, M., Cohen, J. A., Filippi, M., Fujihara, K., Havrdova, E., Hutchinson, M., Kappos, L., Lublin, F. D., Montalban, X., O’Connor, P., Sandberg-Wollheim, M., Thompson, A. J., Waubant, E., Weinshenker, B., & Wolinsky, J. S. (2011). Diagnostic criteria for multiple sclerosis: 2010 revisions to the McDonald criteria. Annals of Neurology, 69(2), 292–302. [Link]
- McDonald, W. I., Compston, A., Edan, G., Goodkin, D., Hartung, H. P., Lublin, F. D., McFarland, H. F., Paty, D. W., Polman, C. H., Reingold, S. C., Sandberg-Wollheim, M., Sibley, W., Thompson, A., van den Noort, S., Weinshenker, B. Y., & Wolinsky, J. S. (2001). Recommended diagnostic criteria for multiple sclerosis: Guidelines from the International Panel on the diagnosis of multiple sclerosis. Annals of Neurology, 50(1), 121–127. [Link]
- Polman, C. H., Reingold, S. C., Edan, G., Filippi, M., Hartung, H. P., Kappos, L., Lublin, F. D., Metz, L. M., McFarland, H. F., O’Connor, P. W., Sandberg-Wollheim, M., Thompson, A. J., Weinshenker, B. G., & Wolinsky, J. S. (2005). Diagnostic criteria for multiple sclerosis: 2005 revisions to the “McDonald Criteria”. Annals of neurology, 58(6), 840–846. [Link]
- Thompson, A. J., Banwell, B. L., Barkhof, F., Carroll, W. M., Coetzee, T., Comi, G., Correale, J., Fazekas, F., Filippi, M., Freedman, M. S., Fujihara, K., Galetta, S. L., Hartung, H. P., Kappos, L., Lublin, F. D., Marrie, R. A., Miller, A. E., Miller, D. H., Montalban, X., Mowry, E. M., … Cohen, J. A. (2018). Diagnosis of multiple sclerosis: 2017 revisions of the McDonald criteria. The Lancet. Neurology, 17(2), 162–173. [Link]
- Rovira, À., Wattjes, M. P., Tintoré, M., Tur, C., Yousry, T. A., Sormani, M. P., De Stefano, N., Filippi, M., Auger, C., Rocca, M. A., Barkhof, F., Fazekas, F., Kappos, L., Polman, C., Miller, D., Montalban, X., & MAGNIMS study group (2015). Evidence-based guidelines: MAGNIMS consensus guidelines on the use of MRI in multiple sclerosis-clinical implementation in the diagnostic process. Nature Reviews. Neurology, 11(8), 471–482. [Link]
- Traboulsee, A., Simon, J. H., Stone, L., Fisher, E., Jones, D. E., Malhotra, A., Newsome, S. D., Oh, J., Reich, D. S., Richert, N., Rammohan, K., Khan, O., Radue, E. W., Ford, C., Halper, J., & Li, D. (2016). Revised Recommendations of the Consortium of MS Centers Task Force for a Standardized MRI Protocol and Clinical Guidelines for the Diagnosis and Follow-Up of Multiple Sclerosis. AJNR. American Journal of Neuroradiology, 37(3), 394–401. [Link]
- Wattjes, M. P., Ciccarelli, O., Reich, D. S., Banwell, B., de Stefano, N., Enzinger, C., Fazekas, F., Filippi, M., Frederiksen, J., Gasperini, C., Hacohen, Y., Kappos, L., Li, D., Mankad, K., Montalban, X., Newsome, S. D., Oh, J., Palace, J., Rocca, M. A., Sastre-Garriga, J., … North American Imaging in Multiple Sclerosis Cooperative MRI guidelines working group (2021). 2021 MAGNIMS-CMSC-NAIMS consensus recommendations on the use of MRI in patients with multiple sclerosis. The Lancet. Neurology, 20(8), 653–670. [Link]
- Hannoun, S., Heidelberg, D., Hourani, R., Nguyen, T., Brisset, J. C., Grand, S., Kremer, S., Bonneville, F., Guttmann, C., Dousset, V., Cotton, F., & Imaging Working Group of the Observatoire Français de la Sclérose en Plaques (2018). Diagnostic value of 3DFLAIR in clinical practice for the detection of infratentorial lesions in multiple sclerosis in regard to dual echo T2 sequences. European Journal of Radiology, 102, 146–151. [Link]
- Hannoun, S., Baalbaki, M., Haddad, R., Saaybi, S., El Ayoubi, N. K., Yamout, B. I., Khoury, S. J., & Hourani, R. (2018). Gadolinium effect on thalamus and whole brain tissue segmentation. Neuroradiology, 60(11), 1167–1173. [Link]
- Runge, V. M., Ai, T., Hao, D., & Hu, X. (2011). The developmental history of the gadolinium chelates as intravenous contrast media for magnetic resonance. Investigative Radiology, 46(12), 807–816. [Link]
- Idée, J. M., Fretellier, N., Robic, C., & Corot, C. (2014). The role of gadolinium chelates in the mechanism of nephrogenic systemic fibrosis: A critical update. Critical Reviews in Toxicology, 44(10), 895–913. [Link]
- Grobner, T. (2006). Gadolinium–a specific trigger for the development of nephrogenic fibrosing dermopathy and nephrogenic systemic fibrosis? Nephrology, Dialysis, Transplantation: Official Publication of the European Dialysis and Transplant Association – European Renal Association, 21(4), 1104–1108. [Link]
- Kanda, T., Ishii, K., Kawaguchi, H., Kitajima, K., & Takenaka, D. (2014). High signal intensity in the dentate nucleus and globus pallidus on unenhanced T1-weighted MR images: relationship with increasing cumulative dose of a gadolinium-based contrast material. Radiology, 270(3), 834–841. [Link]
- Kanda, T., Osawa, M., Oba, H., Toyoda, K., Kotoku, J., Haruyama, T., Takeshita, K., & Furui, S. (2015). High signal intensity in dentate nucleus on unenhanced T1-weighted MR images: Association with linear versus macrocyclic gadolinium chelate administration. Radiology, 275(3), 803–809. [Link]
- Hannoun, S., Issa, R., El Ayoubi, N. K., Haddad, R., Baalbaki, M., Yamout, B. I., Khoury, S. J., & Hourani, R. (2019). Gadoterate meglumine administration in multiple sclerosis has no effect on the dentate nucleus and the globus pallidus signal intensities. Academic Radiology, 26(10), e284–e291. [Link]
- Jaulent, P., Hannoun, S., Kocevar, G., Rollot, F., Durand-Dubief, F., Vukusic, S., Brisset, J. C., Sappey-Marinier, D., & Cotton, F. (2018). Weekly enhanced T1-weighted MRI with Gadobutrol injections in MS patients: Is there a signal intensity increase in the dentate nucleus and the globus pallidus? European Journal of Radiology, 105, 204–208. [Link]
- Hannoun, S., Kocevar, G., Codjia, P., Maucort-Boulch, D., Cotton, F., Vukusic, S., Durand-Dubief, F., & Sappey-Marinier, D. (2021). Signal intensity evaluation in the dentate nucleus and subcortical gray matter: Effect of several administrations of gadoterate meglumine in multiple sclerosis. Clinical Neuroradiology, 10.1007/s00062-021-00995-6. Advance online publication. [Link]
- Dekkers, I. A., Roos, R., & van der Molen, A. J. (2018). Gadolinium retention after administration of contrast agents based on linear chelators and the recommendations of the European Medicines Agency. European Radiology, 28(4), 1579–1584. [Link]
- Filippi, M., & Rocca, M. A. (2011). MR imaging of multiple sclerosis. Radiology, 259(3), 659–681. [Link]
- Hannoun, S., Bagory, M., Durand-Dubief, F., Ibarrola, D., Comte, J. C., Confavreux, C., Cotton, F., & Sappey-Marinier, D. (2012). Correlation of diffusion and metabolic alterations in different clinical forms of multiple sclerosis. PloS One, 7(3), e32525. [Link]
- Hannoun, S., Durand-Dubief, F., Confavreux, C., Ibarrola, D., Streichenberger, N., Cotton, F., Guttmann, C. R., & Sappey-Marinier, D. (2012). Diffusion tensor-MRI evidence for extra-axonal neuronal degeneration in caudate and thalamic nuclei of patients with multiple sclerosis. AJNR. American Journal of Neuroradiology, 33(7), 1363–1368. [Link]
Disclaimer: The information provided on this website is intended to provide useful information to radiologic technologists. This information should not replace information provided by state, federal, or professional regulatory and authoritative bodies in the radiological technology industry. While Medical Professionals strives to always provide up-to-date and accurate information, laws, regulations, statutes, rules, and requirements may vary from one state to another and may change. Use of this information is entirely voluntary, and users should always refer to official regulatory bodies before acting on information. Users assume the entire risk as to the results of using the information provided, and in no event shall Medical Professionals be held liable for any direct, consequential, incidental or indirect damages suffered in the course of using the information provided. Medical Professionals hereby disclaims any responsibility for the consequences of any action(s) taken by any user as a result of using the information provided. Users hereby agree not to take action against, or seek to hold, or hold liable, Medical Professionals for the user’s use of the information provided.