Intensity-Modulated Radiation Therapy (IMRT) 3D Planning
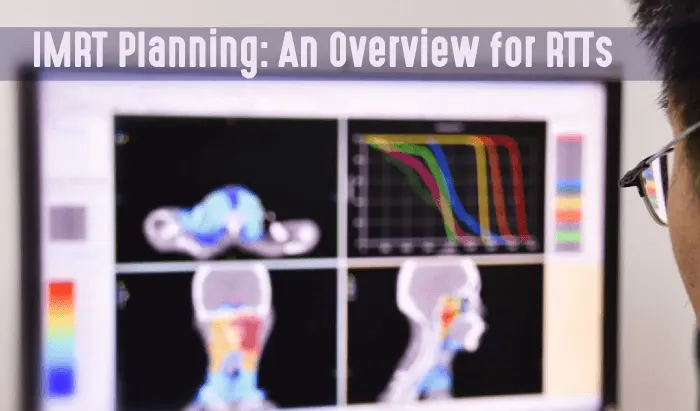
IMRT Planning: An Overview for RTTs on intensity-modulated radiation therapy
An overview for RTTs of IMRT planning, what it is, how it works, and its advantages over conventional conformal 3D planning
This article addresses intensity-modulated radiation therapy (IMRT) planning—specifically the dynamic MLC delivery type—and its importance in radiation therapy treatment. In this article, we’ll define the different delivery types, discuss a method used to compare forward planning and IMRT plans using the DVH and the spatial dose distribution, identify the advantages and disadvantages of these techniques, and discuss their efficiency for patient treatment. We’ll also provide a comparison between forward and inverse plans in order to show the importance of an IMRT plan in different sites of the body including: prostate, head and neck, and breast, since tumors in these sites are common in most countries and tend to be located close to critical organs and tissues in the body.
- What is intensity-modulated radiation therapy (IMRT)?
- IMRT Planning
- IMRT Planning and Case Results
- Final Thoughts
What is intensity-modulated radiation therapy (IMRT)?
The short answer: IMRT is an advanced form of 3D conformal radiotherapy that is particularly valuable for use with target volumes that have complex and concave shapes and are situated close to organs at risk. IMRT has two additional features that exceed the limitations of conformal radiotherapy: the variable intensity of the radiation beams and inverse planning that uses different cost functions to automatically create segments conformed to the targets.
During radiotherapy, dosimetrists should administer a high dose of radiation to the tumor while delivering a low dosage to the surrounding normal tissue for an optimum radiotherapy treatment. There is a definite association between radiation dose and the likelihood of tumor control in complicated cancers, although the tumor dose is frequently limited by the radiation tolerance of nearby tissues. CT scanning has substantially enhanced the precision of defining both the tumor and critical organs. A reconstructed three-dimensional target volume is created by identifying the tumor and drawing it onto each cross-sectional image.
The radiation beams are tailored to fit this volume and offer shielding to the normal tissues using a multi-leaf collimator for 3D conformal advance planning (MLC). Although this technique improves the spatial distribution of the dose, it still has limits, particularly in the case of integrated targets and dose reduction in normal tissues surrounding the tumor. As a result, the more advanced approach — intensity-modulated radiation therapy — is employed to improve coverage and fall off the dose outside the target.
By conforming more precisely to the selected target, IMRT may allow more normal tissue to be spared than with other techniques. IMRT provides the possibility of both reducing late toxicity and increasing the delivered dose, which could lead to improved tumor control and critical organ survival.
The gist: IMRT is an advanced form of 3D conformal radiotherapy that is particularly valuable for use with target volumes that have complex and concave shapes and are situated close to organs at risk.
IMRT Planning
In this section, we’ll describe the current status of IMRT used for treatment, highlight the recent advances in imaging that are incorporated into the IMRT planning process, and emphasize the areas of future research.
What is the difference between forward and inverse planning?
Conformal radiotherapy is forward-planned and reliant on the skills of the treatment planner to decide the number, shape, and orientation of the segments. Inverse planning specifies the plan outcome in terms of the tumor dose and normal structure dose limits, and the computer system adjusts the beam intensities to find a configuration best suited to the desired plan.
- Forward Planning
- 3-dimensional conformal radiation therapy (3D-CRT)
- Inverse Planning
- Intensity-modulated radiation therapy (IMRT)
- Step and Shoot
- Dynamic MLC (dMLC)
- Volumetric-modulated arc therapy (VMAT)
- Dynamic conformal arc therapy (DCAT)
- Intensity-modulated radiation therapy (IMRT)
To deliver Intensity-modulated radiation therapy (IMRT), a variety of approaches have been developed. Multi-leaf collimators are used in the two most frequent approaches of segmental IMRT and dynamic IMRT (MLC). The first MLC system was created in 1948 for the delivery of dynamic conformal therapy and has since become the industry standard on current linear accelerators used now in 2021. Advanced LINAC can provide a high modulated dose delivered to the tumor target with less danger to surrounding tissue, and treatment times are dramatically reduced, giving greater patient comfort and capacity. Leaves are typically 5 mm broad and organized in opposing pairs, which can be positioned under computer control to generate an irregular field that conforms to the tumor shape while shielding normal tissues. IMRT can be delivered using 2 techniques: the Step and Shoot and Dynamic MLC.
- Step and Shoot
- This method is the simplest in the inverse planning in radiotherapy. They are different beams from different angles and for each orientation, several different segments are created. The total dose distribution is calculated by summing the dose of the different angles using the modulated intensity. The radiation is only turned on when the segments are in position and so this method is known as segmental MLC. This is available from most linear accelerator manufacturers and is the most widely implemented technique.
- Dynamic MLC
- During DMLC treatment, the leaves are in continuous motion for each field. At a fixed beam angle, the leaves move to create the segment shapes conformed to the target volume to produce the desired fluence profile. The leaves can travel with variation of the speed and intensity of radiation to the specified point. This technique can produce more conformal dose distributions than segmental IMRT and is therefore more desirable for complex cases.
The gist: Intensity-modulated radiation therapy (IMRT) in Inverse planning is an advanced technique that uses variable intensity of radiation to obtain homogenous dose distribution inside the target, IMRT is divided into different types called “Step and shoot” and “Dynamic MLC” techniques, (more advanced techniques are used in the inverse planning called VMAT and DCAT to be discussed in upcoming articles)
Intensity-modulated radiation therapy (IMRT) Planning and Case Results
Figure 1: Total Fluence of the 7 beams
An advanced treatment planning system (TPS) was used to compare 3D and IMRT plans of the same tumors. The results showed IMRT planning was of better quality, resulting in improved outcomes for patient treatment.
The TPS can use different algorithms for optimization, segmentation, and final dose calculation. The image is divided into small voxels, each voxel receiving a different dose from different beams. More specifically, each beam is subdivided into hundreds of beamlets, each with its own intensity level, allowing for the creation of an extremely intricate pattern as illustrated in figure 1. Using multiple beams to provide a highly conformal dose distribution allows for precision contouring to a curved target and hence more normal tissue sparing. The high dose region fits the target volume precisely, protecting the centrally situated organs at risk.
An initial dose distribution is created by tracing each beamlet through the subject. Then, a slight modification in the weighting of a single beamlet is made, and, if it improves the distribution, this modification is approved. During a single cycle (iteration), this process is repeated for all beamlets, resulting in a better layout. The iterative process is repeated for many cycles until no further improvement is seen, resulting in optimal intensity across each beam to produce the specified dose distribution. The calculations in this process would be impossible with a forward-planned manual technique.
Many revisions and optimizations are required to develop the plan quality. For this reason, new TPSs include a template that can be saved and used for different patients having the same cancer site. These templates can conserve the beam orientation and number of beams, the sequencing and calculation parameters, the prescription and number of fractions, and finally the cost functions used to make the dose distribution.
The gist: IMRT planning uses iterative cycles of calculation to adjust the intensity of hundreds of beamlets and improve dose distribution to the target volume more precisely while protecting healthy organ tissue
Head and Neck Cancer
Figure 2: Beams orientation for a head and neck case
A head and neck case was planned using the IMRT technique. Three targets were created, each with a different prescription: 66, 60, and 54 Gy. These tumors have great potential to benefit from IMRT because of the complexity and concavity of the target volume and the proximity of radiosensitive dose-limiting structures. A 7-beams plan was created with angles having the characteristics listed in figure 3.
Figure 3: Characteristics of the beams used for head and neck case
Head and Neck Cancer Results
Figure 4: Dose distribution using 7 beams in IMRT planning
The results of the IMRT plan above show that the spared parotid glands recovered to 63% of their pre-treatment level, compared to only a 3% recovery rate in glands treated with conventional doses. A review different patients treated with IMRT showed good local control, with no increase in relapse in the region adjacent to the spared parotid glands. The target doses for the spinal cord (sc), parotid, lymph nodes and tumor are marked. The IMRT plan delivers a high dose (red) to the tumor and low dose (blue) to the contralateral parotid gland.
Figure 5: DVH of the head and neck structures
In addition to dose escalation, nodal irradiation with a boost to the primary tumor is now possible as a single-phase treatment using IMRT. The high-dose region conforms to the target volume in a concave shape reducing the dose to the parotids, spinal cord, and brainstem. Figure 5 shows the dose volume histogram (DVH) of all the structures: planning target volume (PTV) 66, PTV 60, PTV 54, (parotids, spinal cord, and brainstem). The PTVs are very well covered (more than 98% of the volume is covered by 95% of the prescription dose) with the different prescriptions and the parotids are protected from high doses. And we can see clearly the overlapping region between the parotid and PTV54 in figure 5.
Prostate Cancer
Here, we investigate a comparison between a 3D conformal conventional plan and an IMRT plan. A 3D plan was prepared using 7 beams conformed to the PTV having the gantry angles as presented in figure 6.
Figure 6: 7 beams conformed to PTV
And the IMRT plan was prepared using 7 beams having the following characteristics:
Figure 7: And the IMRT plan was prepared using 7 beams having the following characteristics
Prostate Cancer Results
The dosimetric benefit is assessed using a comparative dose-volume histogram that shows the volume of bladder irradiated when the patient is treated with conventional, conformal planning (figure 10) and IMRT planning (figure 11). The volume receiving more than 40 Gy is reduced by 20% using conformal radiotherapy, and there is a further 45% reduction using IMRT. In addition, the 40% isoline inside the patient was reduced, as clearly appears in the reduction of the blue isolines between figures 8 and 9.
![]() ![]() |
![]() ![]()
|
The DHV demonstrates the dramatic improvement in dosimetry that can be achieved with this technique: the low dose in the patient was reduced to a volume of 17 % for a dose of 17 Gy, the rectum and bladder were more protected, the maximum dose was reduced in the PTV, and the coverage was also improved.
Breast Cancer
Post-operative radiotherapy for breast cancer gives significant benefits in local control and survival, but long-term follow up studies have shown increased side effects including cardiac disease, lung damage, breast distortion, rib fractures, and pain. IMRT can improve the dose uniformity by compensating for missing tissue and can, consequently, improve the cosmetic outcome. It is possible to treat using two beams in order to compare an IMRT plan with a 3D conventional plan. Two additional beams can be added to improve the coverage beams with two tangents and two other beams with an angle of 15 degrees.
Figure 12: Two tangential beams for a breast case
In this study, only two beams are used to compare IMRT with 3D conventional planning, with the MLC beams having the characteristics shown in figure 13.
Figure 13: Characteristics of the beams used for a breast cancer
Breast Cancer Results
The results in figures 14 and 15 show that the dose distribution using a forward plan presents some hotspots of about 106% of the prescription. The patient’s lung also received dose, especially at the zones near the PTV. On the other hand, the IMRT plan resulted in a homogenous distribution of the dose inside the PTV, while the maximum dose was reduced to 102% of the prescription and the lung was more protected, with a dose of 20cGy covering less than 10% of the lung.
Figure 14: Dose distribution of the 3D plan
Figure 15: Dose distribution of the IMRT plan
Figures 16 and 17 show the differences between the forward plan and the IMRT plan. The coverage is improved using the IMRT technique, and the dose inside the left lung is reduced to better protect this critical organ.
Figure 16: DVH of the 3D plan
Figure 17: DVH of the IMRT plan
Advantages and Disadvantages of IMRT Planning
Advantages
IMRT improves the target conformity, particularly for concave target volumes. It produces intentional dose inhomogeneity, and enables dose escalation within any gross tumor volume to define one or more target volumes and to deliver a higher dose to these regions. In addition, IMRT Increases normal tissue sparing. Noncoplanar beams can provide more freedom in the beam arrangement to achieve good conformity, avoid critical structures, enable fast dose fall-off outside the target, and compensate for missing tissue.
Disadvantages
On the other hand, IMRT can increase clinician time for target and organ outlining. It requires an extensive quality assurance program to verify the positioning and the dose delivered to the phantom before the treatment of any patient. In addition, it increases machine treatment time, as well as total body irradiation.
Another technique called volumetric modulated radiation therapy (VMAT) is used to avoid the limitations of IMRT planning. VMAT uses a process that works using a continuous arc instead of separate fields, thereby allowing irradiation with simultaneously varying dose rate, gantry speed, collimator, and leaf positions. VMAT prescribes the dose inside the target for each voxel as the function of the signal volumetric intensity of that voxel in the biologic image to reduce the delivery time and to protect at maximum the organ at risk and the patient from low doses. This technique will be described in detail in an upcoming article in this series (stay tuned!).
The gist: While IMRT allows for greater beam freedom that results in better conformity, protection of normal tissue, and faster dose fall-off outside the target, it takes clinicians more time to plan and outline the target and organs and increases machine treatment times and total body irradiation low dose. Extensive QA programs are a must for IMRT
Final Thoughts
Intensity-modulated radiation therapy is a rapidly developing the field of research. It represents one of the most important advances in radiotherapy, and it is still being evaluated with different TPS calculation methods using the Monte Carlo algorithm. There are many published reports of comparative planning studies that demonstrate the superior dose distribution that can be achieved using IMRT planning for various tumor sites, including prostate, breast, glioma, medulloblastoma, gynecological, and head and neck tumors. IMRT offers the potential to improve clinical outcomes and reduce morbidity, features that are not normally associated with previous 3D forward-planning techniques.
References
- Cannon, D. M., & Lee, N. Y. (2008). Recurrence in region of spared parotid gland after definitive intensity-modulated radiotherapy for head and neck cancer. International Journal of Radiation Oncology, Biology, Physics, 70(3), 660–665. https://doi.org/
- Chen, A. M., Farwell, D. G., Luu, Q., Chen, L. M., Vijayakumar, S., & Purdy, J. A. (2011). Marginal misses after postoperative intensity-modulated radiotherapy for head and neck cancer. International Journal of Radiation Oncology, Biology, Physics, 80(5), 1423–1429. https://doi.org/
- Damast, S., Wolden, S., & Lee, N. (2012). Marginal recurrences after selective targeting with intensity-modulated radiotherapy for oral tongue cancer. Head & Neck, 34(6), 900–906. https://doi.org/
- Dearnaley, D. P., Khoo, V. S., Norman, A. R., Meyer, L., Nahum, A., Tait, D., Yarnold, J., & Horwich, A. (1999). Comparison of radiation side-effects of conformal and conventional radiotherapy in prostate cancer: a randomised trial. Lancet (London, England), 353(9149), 267–272. https://doi.org/
- Dearnaley, D. P., Sydes, M. R., Graham, J. D., Aird, E. G., Bottomley, D., Cowan, R. A., Huddart, R. A., Jose, C. C., Matthews, J. H., Millar, J., Moore, A. R., Morgan, R. C., Russell, J. M., Scrase, C. D., Stephens, R. J., Syndikus, I., Parmar, M. K., & RT01 collaborators (2007). Escalated-dose versus standard-dose conformal radiotherapy in prostate cancer: first results from the MRC RT01 randomised controlled trial. The Lancet Oncology, 8(6), 475–487. https://doi.org/
- Dobler, B., Weidner, K. & Koelbl, O. (2015). Application of volumetric modulated arc therapy (VMAT) in a dual-vendor environment. Radiation Oncology, 5, 95. https://doi.org/
- Emami, B., Sethi, A., & Petruzzelli, G. J. (2003). Influence of MRI on target volume delineation and IMRT planning in nasopharyngeal carcinoma. International Journal of Radiation Oncology, Biology, Physics, 57(2), 481–488. https://doi.org/
- Galvin, J. M., & De Neve, W. (2007). Intensity modulating and other radiation therapy devices for dose painting. Journal of Clinical Oncology, 25(8), 924–930. https://doi.org/
- Hanks, G. E., Hanlon, A. L., Epstein, B., & Horwitz, E. M. (2002). Dose response in prostate cancer with 8-12 years’ follow-up. International Journal of Radiation Oncology, Biology, Physics, 54(2), 427–435. https://doi.org/
- Hartford, A. C., Galvin, J. M., Beyer, D. C., Eichler, T. J., Ibbott, G. S., Kavanagh, B., Schultz, C. J., Rosenthal, S. A., American College of Radiology, & American Society for Radiation Oncology. (2012). American College of Radiology (ACR) and American Society for Radiation Oncology (ASTRO) Practice Guideline for Intensity-modulated Radiation Therapy (IMRT). American Journal of Clinical Oncology, 35(6), 612–617. https://doi.org/
- Hodapp, N. Der ICRU-Report 83: Verordnung, Dokumentation und Kommunikation der fluenzmodulierten Photonenstrahlentherapie (IMRT). Strahlenther Onkol, 188, 97–100 (2012). https://doi.org/
- Intensity Modulated Radiation Therapy Collaborative Working Group. (2001). Intensity-modulated radiotherapy: current status and issues of interest. International Journal of Radiation Oncology, Biology, Physics, 51(4), 880–914. https://doi.org/
- Mendenhall, W. M., Amdur, R. J., & Palta, J. R. (2006). Intensity-modulated radiotherapy in the standard management of head and neck cancer: promises and pitfalls. Journal of Clinical Oncology, 24(17), 2618–2623. https://doi.org/
- Miller, K. D., Siegel, R. L., Lin, C. C., Mariotto, A. B., Kramer, J. L., Rowland, J. H., Stein, K. D., Alteri, R., & Jemal, A. (2016). Cancer treatment and survivorship statistics, 2016. CA: A Cancer Journal for Clinicians, 66(4), 271–289. https://doi.org/
- Peeters, S. T., Heemsbergen, W. D., Koper, P. C., van Putten, W. L., Slot, A., Dielwart, M. F., Bonfrer, J. M., Incrocci, L., & Lebesque, J. V. (2006). Dose-response in radiotherapy for localized prostate cancer: results of the Dutch multicenter randomized phase III trial comparing 68 Gy of radiotherapy with 78 Gy. Journal of Clinical Oncology, 24(13), 1990–1996. https://doi.org/
Disclaimer: The information provided on this website is intended to provide useful information to radiologic technologists. This information should not replace information provided by state, federal, or professional regulatory and authoritative bodies in the radiological technology industry. While Medical Professionals strives to always provide up-to-date and accurate information, laws, regulations, statutes, rules, and requirements may vary from one state to another and may change. Use of this information is entirely voluntary, and users should always refer to official regulatory bodies before acting on information. Users assume the entire risk as to the results of using the information provided, and in no event shall Medical Professionals be held liable for any direct, consequential, incidental or indirect damages suffered in the course of using the information provided. Medical Professionals hereby disclaims any responsibility for the consequences of any action(s) taken by any user as a result of using the information provided. Users hereby agree not to take action against, or seek to hold, or hold liable, Medical Professionals for the user’s use of the information provided.